Light conduction through optical fibers has now changed many areas of life enormously. Examples include telecommunications, process technology and spectroscopy, fiber optic sensors and medical diagnostics via catheters.
Laser technology is also an important area, where fiber lasers are gradually replacing other types of lasers. Examples of all of these applications can be found elsewhere in this journal.
A typical optical fiber consists of a core fiber surrounded by a cladding with a lower refractive index than the core. The light is guided in the core by total reflection.
Another property of the fibers is used in laser technology. If a quartz fiber core is doped with ions, it can amplify light and thus be used as an active laser medium.
The most common dopings with typical emission lines are:
Erbium (1550nm), Ytterbium (1030nm), Neodymium (1064nm) and Thullium (1500nm). Double-cladding fibers have established themselves for efficiently coupling the pump light from high-power diodes into a doped fiber.
Here the pump light runs along the fiber in an additional jacket around the core. In this way, a long interaction distance is achieved for efficient pumping of the core fiber.
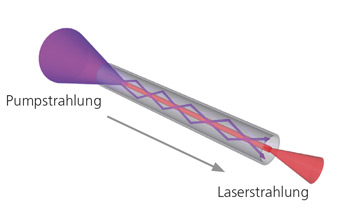
fiber laser
A conventional solid-state laser consists of a pump diode, laser crystal and mirror resonator. If you replace the laser crystal with a doped fiber and the mirrors with Fiber Bragg Gratings (FBG) inserted into the fiber, which selectively reflect the laser wavelength in the core fiber, you get the fiber laser oscillator. The advantages are:
- High stability as the mirrors no longer need to be adjusted
- high gain due to the long interaction distance along the fiber. Up to 70% of the pump diode light will be in
transmit diffraction-limited laser light.
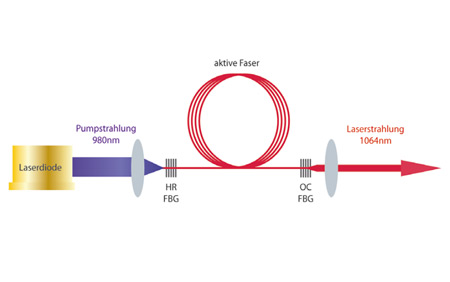
Fiber amplifier
When people talk about fiber lasers today, they often mean a combination of seed laser and several fiber laser amplifiers, so-called EDFAs or YDFAs.
These amplify the output signal of a laser oscillator in several stages. The achievable laser power is limited by the damage threshold of the power amplifier fiber. This is typically 10kW for single-mode fibers and 50kW for multimode fibers.
Because of the high gain in the fibers, feedback must be prevented. Faraday isolators are usually used for this purpose.
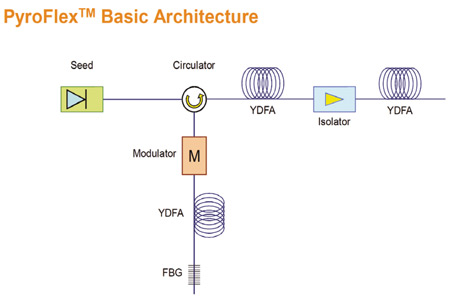
Continuous wave fiber laser
From a user perspective, we differentiate between high-power lasers for material processing (metal cutting, welding, etc.) and low-power lasers for various applications, e.g. in telecommunications or biotechnology.
In the high-performance sector, the company IPG was recently able to demonstrate a fiber laser with 18kW beam power. The frequency-doubled fiber lasers from MBP Communications are becoming increasingly interesting for applications in biotechnology, as many colors in the visible range with sufficient power are now available.
Often several wavelengths in the visible range are specifically required, e.g. for flow cytometry or fluorescence microscopy, in order to excite the various fluorescence markers.
In addition to classic diode lasers, fiber lasers are increasingly being used here, the output of which can be efficiently frequency-doubled using periodically poled crystals, so-called PPLNs. With this technology, the fiber lasers from MBP Communications now achieve significantly higher outputs of 1-5W than corresponding diode lasers.
Pulsed fiber lasers and MOPAs
For pulse operation, fiber lasers are used mode-locked (50fsec-50psec), Q-switched (10-500nsec) or as MOPA (Master Oscillator Power Amplifier). Compact, maintenance-free, mode-locked fiber lasers with 50-100fsec and 100mW can be found, for example, in our time-domain THz spectrometers.
The company Raydiance has introduced the first short-pulse fiber laser with 700fsec and 5W for industrial applications. MOPA designs are slowly becoming established in the nanosecond range for material processing. Here the output of a seed laser (laser diode, solid-state laser or fiber laser) is highly amplified in several fiber amplifiers. This concept offers an invaluable advantage over classic Q-switched lasers. Their pulse duration is determined by the gain in the resonator and therefore varies greatly with the repetition rate, typically between 10-300nsec.
In the MOPA, the seed laser defines the pulse duration and the fiber amplifiers deliver the energy without changing the pulse duration. The seed laser can be a laser diode whose pulse duration is adjustable. However, as shown in the figure above, it can also be a cw diode coupled with a fast electro-optical switch. This cuts out variable pieces from the cw signal, which are then amplified in the fibers.
In this concept, realized by the company ESI-PyroPhotonics Lasers, the transmission of the modulator can also be controlled and various pulse trains can be generated, including multiple pulses with any delays in between.
The pulse train, once set, can then be called up via an external trigger and maintains its shape regardless of the selected repetition rate, in the case of the Pyrophotonics laser up to 500kHz. This property is very valuable for optimizing certain laser processes in material processing.
Limitations of fiber lasers
Three characteristics must be carefully considered when designing fiber lasers to ensure reliable operation:
- the damage threshold of the power amplifier
- the high ASE (attenuated spontaneous emission) due to the high gain
- non-linear effects in the fiber such as SRS (stimulated Raman scattering), SBS (stimulated Brillouin scattering) and SPM (self phase modulation).
ASE can be easily controlled through appropriate measures. Nonlinear effects increase significantly with peak pulse power and affect pulsed applications